Quantum Physics is Cool! Turning water into ice in the quantum realm
- Ken Ecott
- Aug 3, 2019
- 3 min read
Turning liquid water to ice is a piece of cake in our everyday lives. But, on a quantum scale, it requires much more effort.
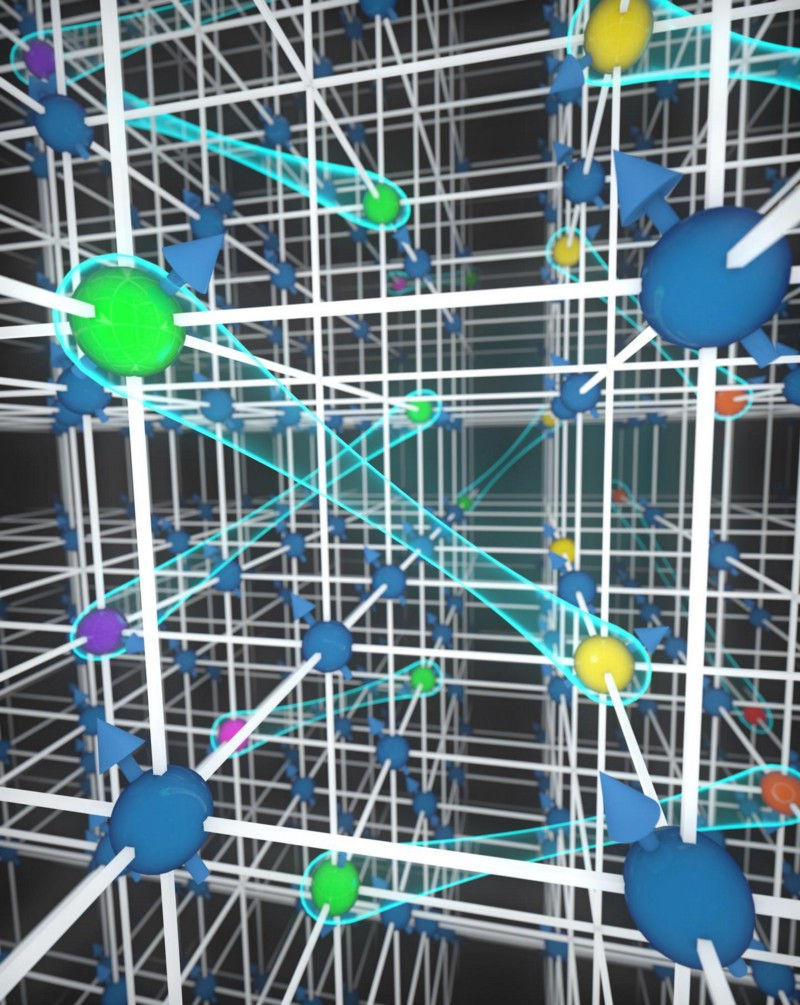
Graphic depicting the weak interactions between neutral atoms in an ultracold gas. (Steven Burrows/JILA)
Transitions between water and ice are pretty couldn’t be more of an everyday phenomenon in our lives. Especially in scorching hot summer, putting water-filled ice trays into the freezer and pulling out ice-cubes later is frequent, and essential.
So reporting on research that features physicists turning water to ice may not initially sound that exciting. The difference here is, the researchers in question — from the University of Colorado and the University of Toronto — have achieved this familiar transition of state with a cloud of ultracold atoms.
The team discovered that it could nudge these quantum materials to undergo transitions between “dynamical phases” — essentially, jumping between two states in which the atoms behave in completely different ways. The findings are published in a paper in the journal Science Advances.
Study co-author Ana Maria Rey, a fellow at JILA, a joint institute between CU Boulder and the National Institute of Standards and Technology (NIST), says: “This happens abruptly, and it resembles the phase transitions we see in systems like water becoming ice.
“But unlike that tray of ice cubes in the freezer, these phases don’t exist in equilibrium. Instead, atoms are constantly shifting and evolving over time.”
The findings, say the researchers, provide an insight into materials that are hard to investigate in the lab.
Rey explains the practical application of the team’s findings: “If you want to, for example, design a quantum communications system to send signals from one place to another, everything will be out of equilibrium.
“Such dynamics will be the key problem to understand if we want to apply what we know to quantum technologies.”
Fermionic atoms — the wallflowers of the atomic dance
Although scientists have previously observed similar transitions before in ultracold atoms, but only among a few dozen charged atoms, or ions, this new research stands out because of its scale. Rey and her team used clouds made up of tens of thousands of uncharged, or neutral, fermionic atoms.
Fermionic atoms are the introverts of the periodic table of elements, says Rey. They don’t want to share their space with their fellow atoms, which can make them harder to control in cold atom laboratories.
Study co-author Joseph Thywissen, a professor of physics at the University of Toronto, continues: “We were really wandering in a new territory not knowing what we would find.”
In order to navigate this new territory, the team used the weak interactions that occur between neutral atoms when they collide in a confined space.
Thywissen and his team in Canada cooled a gas made up of neutral potassium atoms to just a fraction of a degree below absolute zero. They then turned the atoms so that their “spins” had the same orientation.
Spins is a quantum property which isn’t related to angular momentum as you may expect but instead seems to be a magnetic property. Thywissen points out that a particle or atom’s spin has an orientation in a similar way that Earth’s magnetic field — which currently points to the north — does.
Once the atoms were all standing in formation, the group then tweaked them to change how strongly they interacted with each other. And that’s where the fun began.
Thywissem explains: “We ran the experiment using one kind of magnetic field, and the atoms danced in one way.
“Later, we ran the experiment again with a different magnetic field, and the atoms danced in a completely different way.”
Synchronising the atomic dance
In the first dance — or when the atoms barely interacted at all — these particles fell into chaos. The atomic spins began to rotate at their own rates and quickly all pointed in different directions.
The researchers compare this to standing in a room filled with thousands of clocks with second hands all ticking at different tempos.
When the group increased the strength of the interactions between atoms, however, they stopped acting like disordered individuals and more like a collective.
Their spins still ticked, but now they ticked in sync.
In this synchronous phase, the atoms are no longer independent, explains Peiru He, a graduate student in physics at CU Boulder and one of the lead authors of the new paper.
He continues: “They feel each other, and the interactions will drive them to align with each other.”
With the right tweaks, the group also discovered that it could do something else: revert both the synchronized and disordered phases back to their initial state.
In the end, the researchers were only able to maintain those two different dynamical phases of matter for about 0.2 seconds. If they can increase that time, He said, they may be able to make even more interesting observations.
He concludes: “In order to see richer physics, we probably have to wait longer.”
コメント