Could We Take Lessons from Biological Systems to Build a Space Elevator
- Ken Ecott
- Sep 8, 2018
- 6 min read
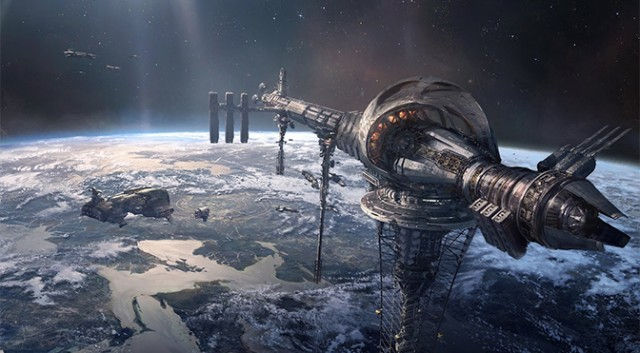
One of the biggest perceived challenges in building megastructures, such as the space elevator, is the unavailability of materials with sufficient tensile strength.
The human desire to create ever bigger and more impressive structures is insatiable. The pyramids of Ancient Egypt, the Great Wall of China and the Burj Khalifa in Dubai – now the tallest edifice in the world at over 828 metres (2,722 ft) – are a consequence of pushing engineering to its limits. But huge buildings aren’t just monuments to human ambition: they might also hold the key to humanity’s progress in the space-faring age.
For more than a century, the scientific community has kicked around the idea of space elevators. Specifics vary, but the basic design involves a vehicle of some sort that travels along a cable that spans from the Earth all the way up into space.
So far, space elevators have been little more than a sci-fi pipe dream. But now researchers from Japan’s Shizuoka University appear determined to bring the concept to reality — they’re ready to conduct the first test of elevator movement in space.
Such a tower would be an alternative to rocket-based transport, and drastically reduce the amount of energy it takes to get into space. Beyond that, we can imagine space-based megastructures many kilometres in size, powered by solar energy, perhaps encompassing whole planets or even stars.
In recent years, engineers have been able to build on grander scales thanks to the strength and reliability of substances such as novel steel alloys. But as we enter the realm of megastructures – those of 1,000 km or more in dimension – maintaining safety and structural integrity has become a fiendish challenge. That’s because the bigger something becomes, the more stress it experiences due to its weight and size. (‘Stress’ is a measure of mechanical tension, like when you pull something apart from either end, or squeeze it together. ‘Strength’ is the maximum tension a structure can withstand before it breaks.)
There's no theoretical reason why the space elevator can't work. But the strength and weight (density) requirements for the primary construction material are very onerous. Carbon nanotubes and a few other cutting-edge exotic materials show the most promise right now. However, at present,we can't produce these materials in large enough (read: industrially useful) quantities.
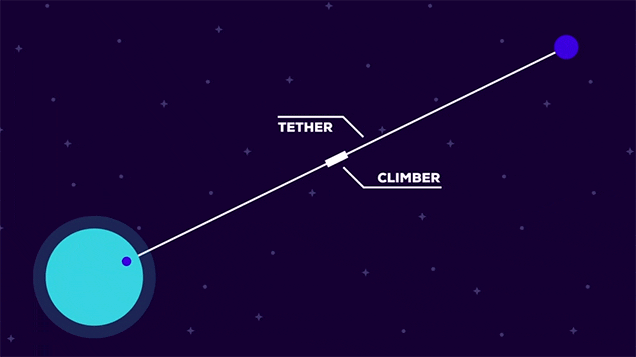
Imagine a ribbon roughly one hundred million times as long as it is wide. If it were a meter long, it would be 10 nanometers wide, or just a few times thicker than a DNA double helix. Scaled up to the length of a football field, it would still be less than a micrometer across — smaller than a red blood cell. Would you trust your life to that thread? What about a tether 100,000 kilometers long, one stretching from the surface of the Earth to well past geostationary orbit (GEO, 22,236 miles up), but which was still somehow narrower than your own wingspan?
The idea of climbing such a ribbon with just your body weight sounds precarious enough, but the ribbon predicted by a new report from the International Academy of Astronautics (IAA) will be able to carry up to seven 20-ton payloads at once. It will serve as a tether stretching far beyond geostationary (aka geosynchronous) orbit and held taught by an anchor of roughly two million kilograms. Sending payloads up this backbone could fundamentally change the human relationship with space — every climber sent up the tether could match the space shuttle in capacity, allowing up to a “launch” every couple of days.
Currently the tallest building in the world is the Burj Khalifa in Dubai, Standing proudly at 829.8m. Holding the record as the tallest structure ever built by mankind, surpassing the previous record holder, the Warsaw Radio Mast 646.38m, which actually collapsed during its construction. A feasible space elevator would have to be at minimum 35,786km tall - the height at which an object reaches Geostationary Orbit around the Earth. For comparison, if the Burj Khalifa was the height of a coffee cup 8.25cm, then a Space Elevator would be 3.56km tall - over 4.3 times the height of the actual Burg Khalifa. Thats a mind boggling leap in construction.
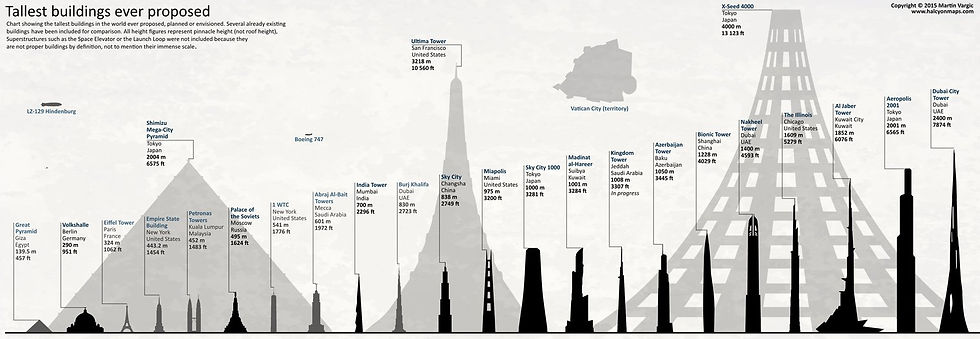
It turns out that biological design, equipped with around 3.8 billion years of experience, might help solve this puzzle. Before the age of materials science, engineers had to look to nature for creative tricks to help them overcome the restrictions of their materials. Classical civilisations, for example, souped their war machines with twisted tendons made from animal hides, which could extend and snap back to launch projectiles at the enemy. But then substances such as steel and concrete arrived, and became successively tougher and lighter.
This led to a sub-discipline known as ‘reliability engineering’. Designers started to make structures that were much stronger than the maximum possible load they needed to bear – which meant the stress on the materials stayed within a range where the probability of breakage was very low. Once structures turn into megastructures, though, calculations show that this risk-averse approach places a cap on their size. Megastructures necessarily push materials to their limits, and remove the luxury of weathering comfortable levels of stress.
However, neither the bones nor tendons in our bodies enjoy this luxury. In fact, they’re often compressed and stretched well beyond the point at which their underlying substances might be expected to break. Yet these components of human bodies are still much more ‘reliable’ than their sheer material strength would suggest. For example, merely running can push the Achilles’ tendon to over 75 per cent of its ultimate tensile strength, whereas weightlifters can experience stresses of over 90 per cent of the strength of their lumbar spines, when they are hefting hundreds of kilogrammes.
How does biology handle these loads? The answer is that our bodies constantly repair and recycle their materials. In tendons, collagen fibres are replaced in such a way that, while some are damaged, the overall tendon is safe. This constant self-repair is efficient and inexpensive, and can change based on the load. Indeed, all structures and cells in our bodies are in constant turnover; it’s estimated that almost 98 per cent of the atoms in the human body are replaced every year.
We recently applied this self-repair paradigm to see whether it’s possible to build a reliable space elevator with available materials. A common proposed design features a 91,000 km-long cable (called a tether), extending out from the Equator and balanced by a counterweight in space. The tether would consist of bundles of parallel fibres, similar to collagen fibres in tendons or osteons in bones, but made from Kevlar, a material found in bullet-proof and knife-proof vests. Using sensors and artificially intelligent software, it would be possible to model the whole tether mathematically so as to predict when, where and how the fibres would break. And when they did, speedy robotic climbers patrolling up and down the tether would replace them, adjusting the rate of maintenance and repair as needed – mimicking the sensitivity of biological processes. Despite operating at very high stress compared to what materials can sustain, we showed this structure would be reliable and would not demand exorbitant rates of replacement. Moreover, the maximum strength the material would need to possess to achieve a dependable structure was cut by an impressive 44 per cent.
So. Space elevators. Are they are thing that we should talk about? ~Credit: Kurzgesagt – In a Nutshell
This bio-inspired approach to engineering can also help structures down here on Earth, such as bridges and skyscrapers. By ‘challenging’ our materials, and equipping systems with autonomous repair and replacement mechanisms, we can exceed current limitations while improving reliability. To get a sense of the benefits of operating closer to the limit of tensile strength, look at a suspension bridge, involving lengths of steel rope that dip in the middle. The main hurdle to increasing the span of the bridge is that, as we use longer ropes, they become heavier and break under their own weight. If the rope is stretched to no more than 50 per cent of its total strength, the maximum span is about 4 km; but when stretched up to 90 per cent of its strength, the span dramatically increases to more than 7.5 km. However, ensuring the cable is safe will require steel fibres to be replaced in a fine-tuned process, just like in biological systems.
Megastructures are no longer science fiction. Never dissuaded by the collapse of the Tower of Babel, as recounted in the Old Testament, humans have continued to build bigger and higher and faster, fuelled by tremendous advances in science and technology. Yet according to the standards of classical reliability engineering, we are still far away. Instead we need a new paradigm, one that focuses not only on material strength, but on systems’ inherent reconstructive capacities. We ought to look no further than the bounty of biological life around us and trust there is much to learn from the sweep of evolutionary history.
Comments